Soil is the most fundamental resource for the farmer, without which food and natural fibre cannot be produced. This article forms part of a series to highlight this resource.
Soil water management is of cardinal importance in crop production. The purpose of soil water management is to continuously comply with the plant’s water needs as well as one can. Too little water places moisture stress on the plant, lowering the crop yield. Conversely, too much water causes water logging conditions. In this article soil water management will be discussed in terms of the soil water balance equation.
The soil water balance equation
The soil water balance equation can be mathematically described as follows: ΔW = (P+I) – (R±D+E+T)
Where: ∆W = change in soil water content
P | = precipitation / rainfall |
I | = supplementation via irrigation water |
R | = run-off from the soil surface |
D | = deep percolation (drainage past the root zone) or capillary rise from a water table |
E | = evaporation losses from the soil |
T | = Transpiration by plant communities on the soil |
The soil water balance equation is presented schematically in Figure 1.
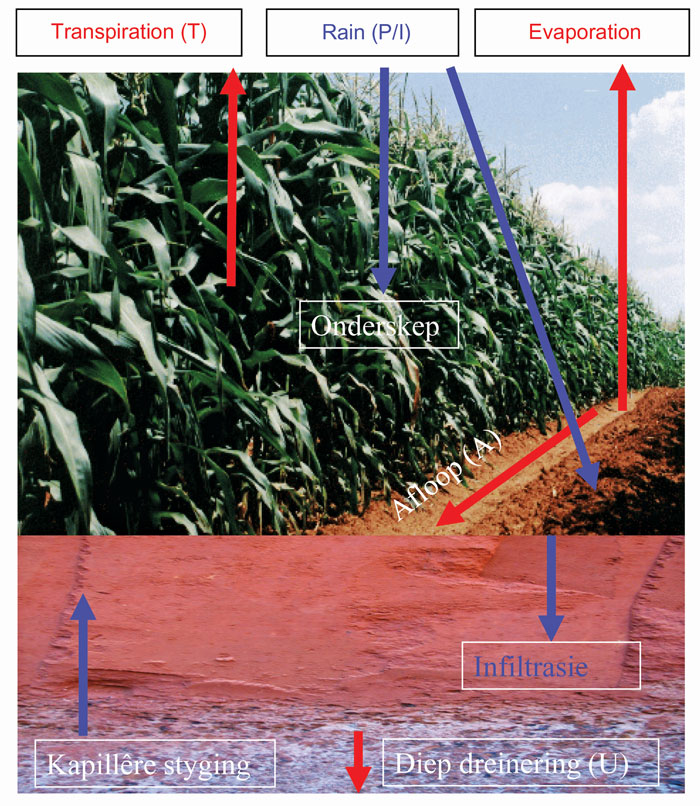
Change in soil water content (∆W)
The soil water content is dynamic over time and changes are brought about by a series of factors. The soil water content is of major importance to the soil user in agriculture because it is probably the greatest single factor that influences plant growth. The dry land producer tries to utilise the physical and morphological properties of soil in such a way that it stores water optimally and makes it available to the plants during non- or low rainfall times. The irrigation producer has the same aim, but with the provision that excess water does not build up in the root zone and the soil then become water logged.
The change in soil water content can be measured in a number of ways, of which some methods are direct and others indirect measurements.
Direct measurements
With the gravimetric method, the wet soil is weighed beforehand, dried, and then weighed again. The difference in mass represents the water that was in the soil. It is then expressed as a fraction of the dried soil (g water /g soil). When the bulk density of the soil is available, it can be expressed as a volume basis (mm water /mm soil) and is then referred to as the volumetric water content. The latter is more preferable as it can easily be converted into the water content of the profile by multiplying it by the depth of the soil.
Indirect measurements
The first method is with the help of a neutron moisture probe. This is based on the principle that a neutron source is lowered into the soil via an access tube. The neutron source radiates rapidly moving neutrons that are slowed down by a number of collisions with hydrogen nuclei (present in water). A counter measures the relationship between the fast and the slow neutrons which is related to the hydrogen and therefore the water content of the soil. It is important that the neutron meter measurements are correctly calibrated with the soil’s water constants, otherwise incorrect readings will be obtained.
Tensiometers measure the matrix potential (suction tension) in the soil and is an indication of the work a plant must perform to withdraw water from the soil. A small ceramic head is connected to a water-filled tube and installed into the soil. A pressure gauge is attached to the top of the tube in order to measure the suction tension when the dry soil sucks the water through the ceramic head.
Nowadays there are a number of electronic appliances on the market that measure the soil water content on a daily basis (even at various depths) and send the data to a computer via SMS or other technology. The change in water content is then presented graphically over time. This is a wonderful aid for irrigation scheduling.
Precipitation / rainfall (P)
For the dry land producer, rainfall is a gift and part of the natural resources that he works with. The challenge is to convert the rainfall into a marketable product, such as grain, fibre, and meat, in the most efficient way. In the soil water balance equation, rainfall is the main source of water that wets the soil and therefore positively changes it. Accumulation of run-off water in low-lying places in fact increases the precipitation in those places. The addition of rain water can be managed by planting in such a way that critical growth periods correspond with periods with the highest likelihood of rainfall.
Addition via irrigation water (I)
Irrigation refers to the artificial addition of water (rainfall is thus excluded) and therefore brings about an increase in the soil water. P + I are the only water gains in the water balance equation. The soil water balance equation can be artificially influenced by farming practices to the advantage or disadvantage of the operations.
Run-off from the soil surface (R)
Run-off is the first loss of water in the water balance equation. It is directly influenced by infiltration. Water that does not infiltrate, remains standing on the surface of the soil or it runs off and is therefore lost for dry land production. Limitation of run-off losses is one of the most important aspects of the water balance equation that can be managed by the producer, in that practices must be focused on increasing water infiltration.
When the topsoil is dry and is then wetted, the initial infiltration is very quick due to the low matrix potential and the water is in fact sucked into the soil. The infiltration rate decreases rapidly as the first pores are filled with water and it must then be conducted deeper. With time the infiltration rate decreases further until it reaches a point where it is constant, which is known as the final infiltration rate of that specific soil (Figure 2). This is equal to the saturated hydraulic conductivity of the soil. In sand this is relatively quick (>100 mm/hour), but in silty and clayey soils this is much slower (<1 mm/hour). When the precipitation exceeds the infiltration rate, run-off will take place.
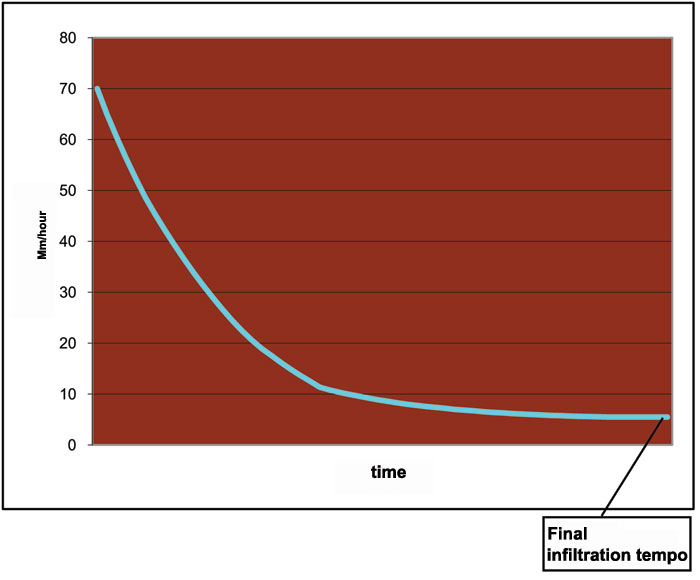
One of the best known methods to increase water infiltration is to loosen the soil surface through mechanical methods, thus making it more receptive to the retention of water. The disadvantage of this is that a large excess of oxygen is introduced into the soil which increases microbe activity and, in turn, breaks down organic material more rapidly. The effect thereof is that micro-aggregates break down, the soil disperses and then closes up, which once again lowers the infiltration and increases the run-off.
The build-up of organic material in the topsoil is intended, amongst others, to increase infiltration. This happens when microbe activity forms water-stable aggregates on the surface, with relatively large pores in between, through which the water can infiltrate rapidly. Furthermore, plant residues on the soil surface reduce the impact of the raindrops and slows down the flow of water, leaving the water more time to infiltrate.
The construction of terraces flattens the terrain (reduces the slope), which reduces runoff by slowing down the overland flow and keeping the water more time on the soil surface, which gaining more time for it to infiltrate. Plots for flood irrigation are constructed in such a way that water runs slow enough over it, leaving enough time for it to infiltrate. Chemical problems can also cause the topsoil to disperse and close up, thus reducing infiltration. The best known is sodium which is associated with the soil becoming brackish. Too much magnesium (>35% of the CEC) has the same effect. Both can be improved with the addition of agricultural gypsum.
Deep percolation or drainage past the root zone (D)
Soil types differ greatly from each other where the ability to retain free water in the profile against deep percolation is concerned or, on the other hand, to allow it to percolate deeply past the root zone. Soil types with restricting layers retain the water against deep percolation and then make it available again to plant roots in the soil, often through capillary rise. The external drainage of these soils is poor. The well-known water-table soils of the Western Free State are perfect examples of this (Figure 3). In the water balance equation, D then has a positive value and is therefore a water profit. These types of soil are usually not the first choice for irrigation.
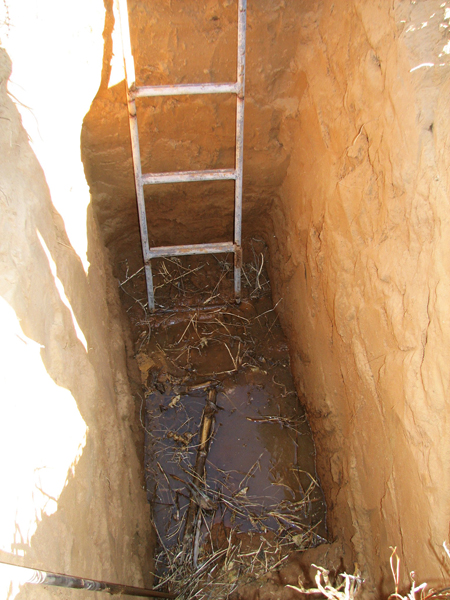
On the other hand, some types of soil have no restricting layers and allows the water to flow through to the underlying geology. These are typically the deep well drained soil types. A significant amount of water can be lost to plant production in these soils. Because it is inherently a soil property, the land user cannot manipulate it. What is of importance though is that the land user must know what types of soil occur on his or her farm and only then the soil types can be utilised according to its drainage properties and full potential.
Evaporation losses from the soil (E)
A significant amount of water evaporates annually from the soil surface. When the soil is hot and dry and a light shower of rain (<5 mm) falls on the soil, it easily evaporates again within a day or two. During a year there are a number of showers like this that, without follow-up showers, the water will evaporate entirely. Also, of each larger shower of rain or rain period, 10 mm easily evaporates. In a fallow land system, when land lies fallow for an entire year in order to accumulate water, about 60% to 70% of the total annual rainfall evaporates. When there is a crop on the land, this is considerably less, but nevertheless between 30% and 50% of the rainwater is lost to evaporation.
Evaporation is one of the factors that can be managed through agricultural practices. Plant residue on the soil surface plays an important role in providing shade for the soil and, as a result, less solar energy falls on the soil thus restricting the evaporation. This role is applicable as long as the soil surface is wet or moist. When the uppermost more or less 100 mm of the soil has already dried out (as is the case during most of the year), the effect of the plant residue and shade is very small, as the rate of water loss is subject to the rate of capillary rise. Under well-drained conditions this supplementation from the underlying layers of soil is very slow, about 0,1 to 0,2 mm water per day. The supplementation of water from shallow water tables (<600 mm from the soil surface) is, however, rapid and therefore the evaporation losses can be significant.
The management of evaporation losses offers the innovative producer major opportunities as better management thereof will have a great influence on yield returns.
Transpiration by plant communities (T)
When the humidity of the atmosphere is lower than in the leaf of the plant (which is normally the case), there is a moisture pressure gradient between the inside of the leaf and the atmosphere. Water moves out of the leaf to the atmosphere (and cools the leaf). The leaf’s moisture state is supplemented again from the soil. When the atmospheric demand is higher than the rate of supplementation from the soil, the plant wilts, it is under stress and photosynthesis is halted.
When the soil is wet, the water is freely available and the plant roots can easily absorb water to provide it to the leaves. The plant remains turgid and photosynthesis is optimal. The drier the atmosphere, the higher the atmospheric evaporative demand and the more the plants transpire. This is often the case in the western grain producing areas of South Africa. In the cooler and moister eastern grain areas the evaporative demand is much lower and the plants use much less water.
Additionally all the water in the soil should only be used by the crop. There should therefore be no unnecessary transpiration losses via weeds. Excellent weed control is therefore the first control measure of the grain producer to restrict transpiration.
Furthermore, the choice of crops and cultivars, as well as plant density, is important. As an example, unproductive shoots amongst maize may be seen as undesirable, as they pump an unnecessary quantity of water into the atmosphere. A plant density which creates too great a leaf area on the land without increasing the harvest returns, is unnecessary and simply increases transpiration.
Transpiration must therefore be managed by the grain farmer in such a way that it optimises the harvest. Unproductive transpiration must also be eliminated.
Interception
When there is a crop on the land and a light shower of rain falls, the plants get wet without much of the rain falling on the soil. This water on the leaves of the plants evaporates rapidly without making a contribution to the water balance equation. This loss is defined as interception. Fortunately, in totality it consists of a small percentage of the total rainfall, but with every shower of rain this loss is there. The producer cannot do much about it.
Summary
A thorough understanding of the water balance equation is of great importance for the producer. Some of the components of the equation can be influenced positively by farming practices and can make a significant contribution to production. The crop farmer have to focus onto those aspects in order thereby enhancing productivity. Efficient management thereof give to the producer the competitive edge for his farming enterprise to be sustainable over the long-term.
In the next article of this series, the uptake of soil water by plant roots will be discussed. For further information, please contact Martiens du Plessis at 072 285 5414 or martiens@nwk.co.za or Prof Cornie van Huyssteen at 051 401 9247 or vanhuysteencw@ufs.ac.za.
References
- Bennie, ATP. 1981 Soil Science 354. Soil and water management. Unpublished class notes for GKD354. University of the Free State: Bloemfontein.
- Bennie, ATP. 1985. SA Co-op, Vol 5, Nr 1.
- Brady, NC. 1990. The nature and properties of soils. 10th ed. Macmillan publishing company: New York.