Soil is the most fundamental resource for the farmer, without which food and natural fibre cannot be produced. This article forms part of a series to highlight this resource.
The movement of water in soil is of great importance in spreading the water throughout the soil profile and making it available to plants. In the previous article the energy state of water in soil was discussed. In this article the soil water constants and the hydraulic flow of water in soil are discussed.
The soil water constants
The amount of water in a soil profile is always changing and yet, by definition, there are a few limits in soil water management which are of great importance to the soil user.
Saturation point
When the soil is excessively wetted through heavy rain or irrigation, it often happens that almost all the air in the pores is forced out and replaced by water. This is regarded as the maximum water capacity of the soil. When this condition takes place over a long period, the soil is regarded as saturated or “water logged”. The matrix water potential = 0 kPa and the osmotic potential is dependent on the salt concentration. The water potential is therefore at its highest. In a well-drained profile the water will flow freely downwards due to the gravitational potential. (FIGURE 1a).
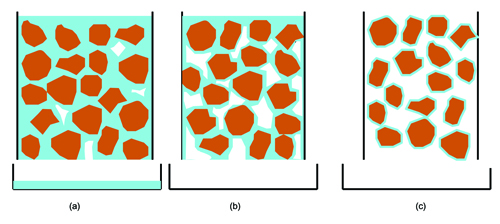
Drained upper limit (DUL) of available plant water (Field water capacity)
Following rain or irrigation the water will, initially move rapidly and later more slowly, downwards due to the gravitational force, until this movement becomes insignificant. In sandy soil, it happens after about two days, in loam after about three days, and after about four to five days in clayey soils. At this point it is said that the soil has reached the drained upper limit (DUL) of available plant water. In the past, the term field (water) capacity was used to describe this.
At this stage the water has drained out of the macro pores and air is once again drawn in from the atmosphere (FIGURE 1b). (By the way, this is the most powerful form of natural soil aeration). The micro and capillary pores are still filled with water. The matrix potential at this point will vary between -10 kPa for sandy soils and -30 kPa for clay soils. For calculation purposes, this point is accepted as the uppermost limit of plant available water. Over time, water will move very slowly, under capillary forces, towards drier parts of the soil profile, with a lower matrix potential.
Lower limit of plant available water (Permanent wilting point)
As the plants take up water out of the soil and water evaporates from the surface of the soil, the matrix potential gradually decreases. At a certain point, the plants cannot extract sufficient water from the soil and begin to wilt during the daytime because the atmospheric demand is higher than the rate of water availability to the plants, but the plants may recover at night. A few days later, the plants will even wilt during the night and at this point the soil is regarded as having reached its lowest level of plant available water (Figure 1c). In the past the term permanent wilting point was used to describe this. The matrix potential is now about -1 500 kPa and the water which is now still in the soil, is present only in the smallest pores and as a very thin layer covering the soil particles. It is no longer available to the majority of plants because the forces holding the water in the soil are too great for the plants to overcome. In sandy soils, there is hardly any water, but it is more significant in clayey soils, even more than what is regarded as plant available water!
Hygroscopic point
When the soil is further dried out by the atmosphere (at 98% humidity), it later comes into balance with the atmosphere and does not dry out any further. The soil moisture content is now at its hygroscopic point with a matrix potential of about -3 000 kPa. Even at this point there is still a quantity of water in the soil. Sandy soils then have very little water, while clay can still hold a significant amount of water. The water is stored in the soil within the clay platelets and is actually not in a liquid state but gaseous.
Plant available water
The water available to plants represents the difference between the drained upper limit and lower limit of plant available water. It differs significantly between soils and can be deduced from the clay content (Table 1).
Table 1: The relationship between the silt + clay content of soil and the soil water constants (Bennie, ATP. 1981)
Silt and clay content (%) | Field water capacity (mm/m) | Permanent wilt point (mm/m) | Plant availability water (mm/m) |
---|---|---|---|
0 - 5 | 70 | 40 | 30 |
10 - 15 | 150 | 50 | 100 |
15 - 20 | 180 | 60 | 120 |
20 - 25 | 200 | 80 | 120 |
25 - 30 | 220 | 100 | 120 |
30 - 40 | 240 | 130 | 110 |
40 - 50 | 260 | 160 | 100 |
50+ | 270 | 200 | 70 |
Types of water movement in the soil
The hydraulic conductivity of a soil is its ability, of water to move under the influence of the driving forces or the soil’s ability to allow water to flow through it. The hydraulic conductivity (or flow) takes place through water-filled pores and along films of water. The hydraulic conductivity is for that reason dependent on the moisture content of the soil. The greater the water content of the soil, the greater the hydraulic conductivity and vice versa. It therefore means that the water flow is highest directly after wetting and thereafter it gradually decreases as the soil dries out. The forces that determine the flow rate are gravity and the matrix potential.
Saturated hydraulic conduction ability
The highest hydraulic conductivity of a soil will be at saturation. A layer of water on top of the soil surface will increase the flow due to a positive matrix or pressure potential. This is illustrated in Figure 2 as the rate at which water flows through the profile, and is measured at the bottom of the profile. The saturated hydraulic conductivity of a soil is an indication of how quickly the soil will drain following heavy rains or irrigation. The higher the saturated hydraulic conductivity of the soil, the quicker excess water will flow through the profile.
Any factor that influences the size and arrangement of the soil pores, will influence the saturated hydraulic conductivity of the soil. In sandy soils, the pores are larger and the resistance to water flow is relatively small, and for that reason the water will flow rapidly through the profile (Figure 3). When sandy soil is compacted, for example by vehicles in a road or via plough compaction, the pores are reduced in size and the flow of the water becomes slower (for example standing water on a farm road after a rain shower). In a clayey soil the pores are smaller (although far greater in number) with the resistance which is now relatively high and again the water will flow more slowly through the profile. Interestingly, is that the flow through a pore with a 1 mm diameter = the flow through 10 000 pores of 0.1 mm. This simply emphasises that the flow rate is mainly determined by the number of larger pores in the soil.
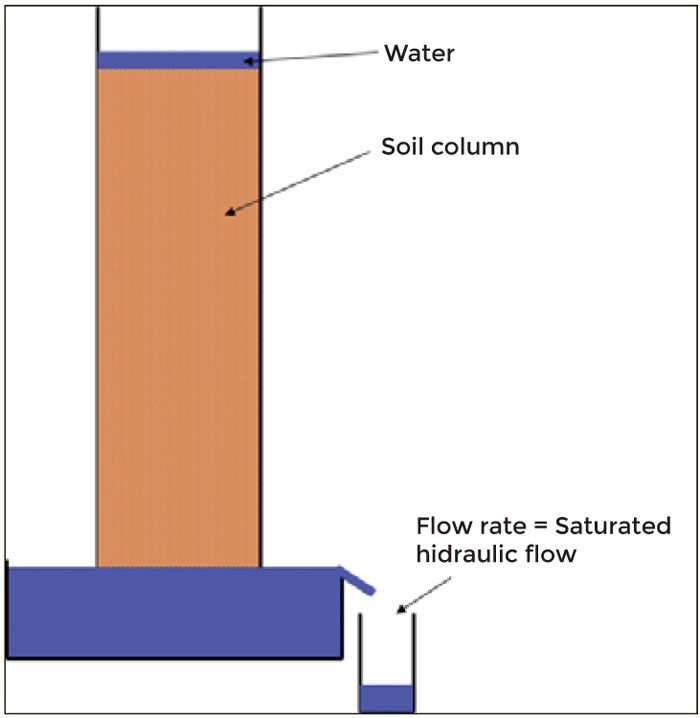
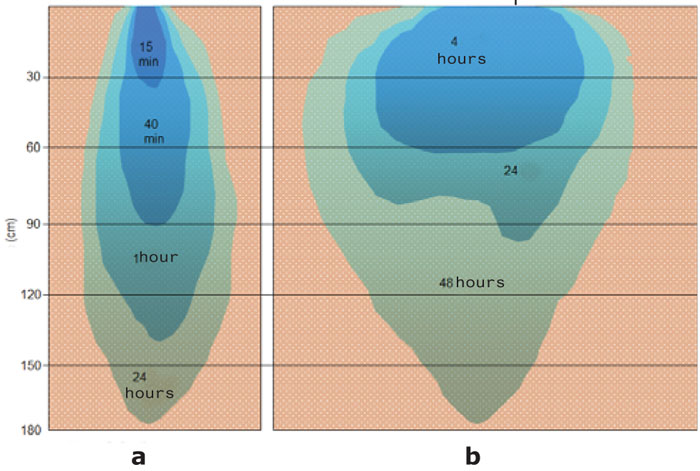
Unsaturated flow
As soon as a soil has been excessively wetted after heavy rain or irrigation and allowed to drain to the drained upper limit, hydraulic conductivity due to gravity is very low and the matrix potential is the dominant driving force. Movement under saturated conditions is rapid and mainly downwards with little lateral movement. Under conditions of unsaturated flow, the water flow is much slower and the movement can be upwards, downwards or laterally. Matrix potential and moisture content differences are now the main driving forces and therefore unsaturated flow becomes increasingly slower as the soil dries out. The unsaturated flow of a soil with a greater matrix potential (like clay soil), is also slower.
The unsaturated flow is of particular importance to the soil user, as it is the main method causing water movement from the wetter parts, further away from the plant root, to the drier parts surrounding the root. In a sandy loam soil, the flow under unsaturated conditions is between 1 and 2 mm per day for a soil which is close to the lower limit. It is a rate that will supply enough water to the plant roots. As the soil gets drier, the flow rate is reduced to a point where water movement is so slow that the water does not reach the plant root quick enough and the plant begins to wilt. In the case of clayey soils, this point will be reached quicker than in sandy soils and drought symptoms are more quickly visible in the clayey soils.
On the drier side of the spectrum the picture is turned around and the flow in the clayey soils keeps on longer than in sandy soils and clayey soil can keep plants alive longer than sand (although under constraint).
Movement of water vapour
Soil pores that are not filled with water are filled with air. Normally the humidity of the soil is about 98% (high vapour pressure). When the soil close to the surface is dry, the humidity there can decrease due to evaporation (lower vapour pressure) and water vapour will move from the higher vapour pressure area (soil) to the lower vapour pressure area (atmosphere). This movement of water vapour in soil is of minimal significance in agriculture. The quantity of water in vapour form is about 15 litres/ha in the upper 15 cm of the soil layer, compared with 375 000 litres/ha water in the liquid form at the lower limit of plant available water.
SUMMARY
Water movement in soil is of extraordinary importance to the farmer, as it is the method that determines the provision of water to plants. Various soil types react differently where water retention and hydraulic conductivity are concerned. With the planting of crops in various soils, the farmer must keep the behaviour of the soil in mind, as it can have a significant impact on the sustainability of the farming enterprise.
In the next article in this series, the behaviour of water in soil will be discussed further. For further information, please contact Martiens du Plessis at 072 285 5414 or martiens@nwk.co.za or Prof Cornie van Huyssteen at 051 401 9247 or vanhuysteencw@ufs.ac.za.
References
- Bennie, ATP. 1981. Soil Science 354. Soil and Water Management. Unpublished class notes for GKD354. University of the Free State: Bloemfontein.
- Bennie, ATP. 1985. SA Coop, Vol. 5, Nr. 1
- Brady, NC. 1990. The nature and properties of soils. 10th ed. Macmillan Publishing Company: New York.
- Brady, NC and Weil, RR. 2002. The nature and properties of soils. 13th ed. Prentice Hall: New Jersey.
- Van Huyssteen, CW. 2009. Soil Ecology. Unpublished class notes for GKD214. University of the Free State: Bloemfontein.