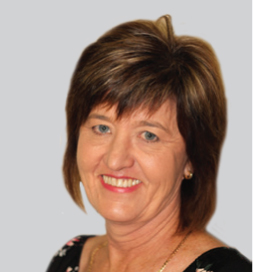
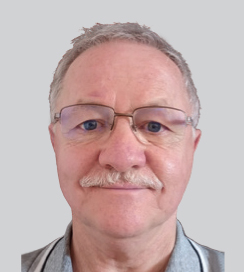
Prof Johnnie van den Berg, Integrated Pest Management programme, North-West University, Potchefstroom
Insecticide application is the most common method used to control insect pests that threaten agricultural production. The long-term use of insecticides results in the evolution of insecticide resistance – globally more than 500 arthropod pest species evolved resistance to insecticides during the last 55 years.
Since the first planting of Bt crops in the world in 1994, eleven target pest species evolved resistance to these plants which produce insecticidal toxins. Many of these resistant species pose serious threats to agricultural production due to the failure of control measures and subsequent yield losses, placing food security in jeopardy.
What is resistance?
The Insecticide Resistance Action Committee (IRAC) defines resistance as a heritable change in the sensitivity of a pest population. Resistance evolves by means of natural selection and mutations resulting in insect populations that evolve mechanisms whereby they can withstand the toxic effects of an insecticide. Producers are usually the first to notice resistance under field conditions when they experience repeated failure of a product to achieve the expected level of control when it is used according to the label recommendation for the target pest species.
Since resistance is a heritable change in sensitivity to a product, it is genetically based, and the evolution thereof is strongly influenced by the genetic makeup of a pest population. The rate of resistance evolution is determined by the initial number of resistant individuals in a pest population, and the factors that determine how strong selection pressure on the population is. The following factors therefore drive the rate of resistance evolution: generation time of the pest, fecundity rate, dispersal ability, frequency of insecticide applications, the dosage applied and persistence of insecticide applications. Since pest populations are usually large and their life cycles short, there is always a risk that insecticide resistance may evolve, especially when insecticides are misused or overused.
The process of resistance evolution after repeated application of an insecticide over many growing seasons is illustrated in Figure 1. In this example, the red beetles indicate individuals in a population that naturally have the genetic traits to survive an insecticide application with a specific mode of action (MoA). It is commonly accepted that there is less than 1 in 1 000 resistant individuals in a population, even before a specific product becomes available for use. When a specific insecticide is continuously applied over seasons, the proportion of insects with the trait that enables it to survive increases compared to the susceptible individuals. The result is then that the population becomes increasingly difficult to control. If this pattern of insecticide application continues, nearly all the individuals within a population become resistant to insecticides with that specific MoA.
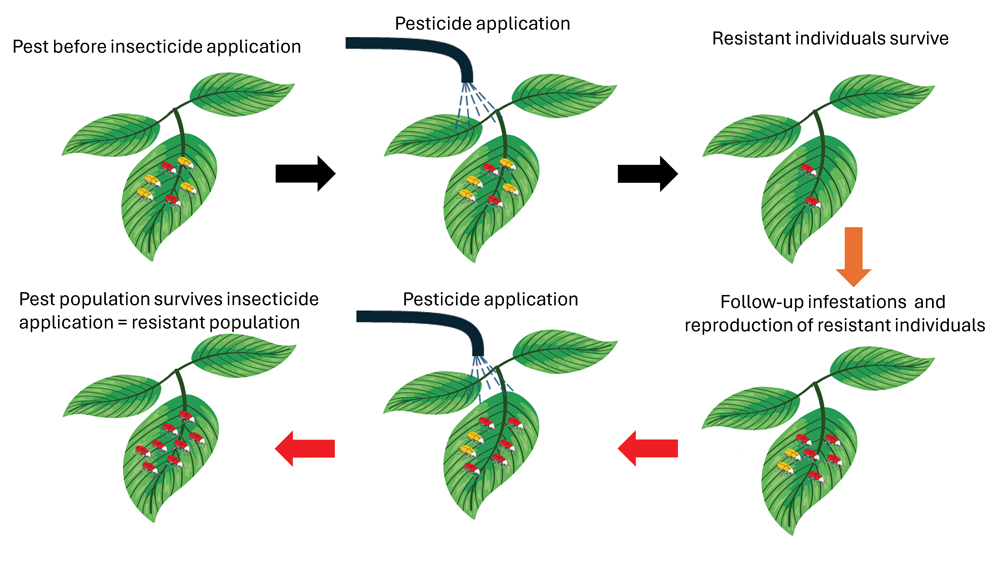
Insecticide resistance mechanisms
The MoA of an insecticide is how it acts at a molecular level within an insect or mite. Within insects, the active ingredients of the insecticide are mobilised by metabolic enzymes and act on target proteins. Therefore, the two major mechanisms of insecticide resistance are metabolic resistance and target site resistance. When metabolic resistance evolves, the production of metabolic enzymes, which detoxify the insecticide, is enhanced within the insect and it is less susceptible to the insecticide. Target site resistance implies that genetic mutations occurred and that the target protein (that was the target of the specific insecticide) has changed, and that the insecticide does not bind at the target site anymore. This causes a loss of sensitivity to the insecticide. Physiological mechanisms may also contribute to reduce insecticidal effects. For example, lower penetration of the chemicals through the insect cuticle, or increased excretion of the insecticide and gut contents of the pest insect after ingestion.
Different chemical classes of insecticides, according to their different biological targets within an insect or mite, have been developed by IRAC (http://www.irac-online.org/documents/moa-classification). Insecticides and miticides are classified according to their MoA. These classes include those acting on the nerves and muscles (neuromuscular system), those targeting the growth of target pests, affecting their cellular respiration, and those acting on the midgut. An example of the latter microbial disruptors of insect midgut membranes, are transgenic crops that express Bacillus thuringiensis (Bt) of Cry toxins. There are also insecticides that are effective in controlling insects by acting on a distinct target site, but their specific MoA is unknown (for example pyridalyl and sulfur).
The class to which a pesticide belongs is indicated on the product label. The use of different classes of insecticides and rotation thereof are important to help prevent or delay the evolution of resistance. The different classes of insecticides form the basis of insecticide resistance management (IRM) programmes (Figure 2).
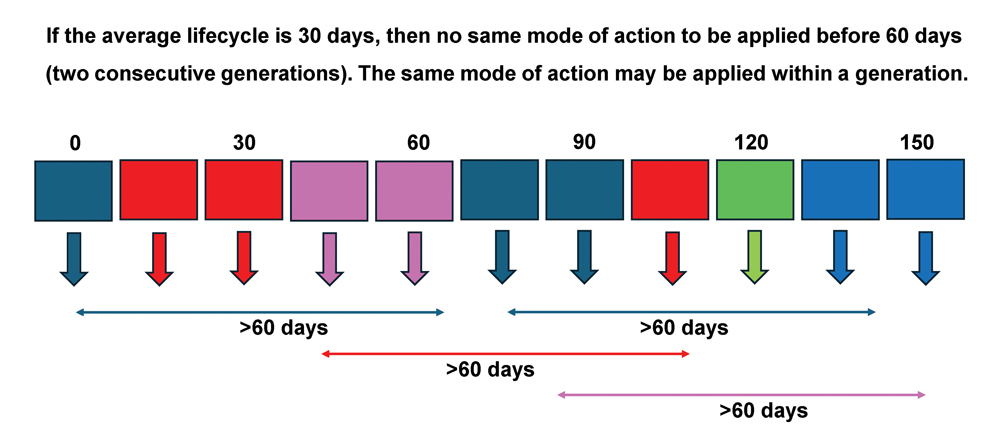
Insecticide resistance management
IRM should be applied to minimise the selection for resistance to any one type of insecticide. Resistance is less likely to occur when insecticides from different MoA classes are applied using sequences or alterations, arranged into MoA spray windows or blocks. These spray windows or blocks should be defined by the stage of crop development and the biology of the pest. One spray window should be equal to the duration of one generation of the target pest. It is important to note that the prevailing environmental temperature determines the rates at which insects develop from the egg to adult stage – development rate increases with increasing temperature. The duration time of one generation will therefore be longer early in the summer production season when temperatures are lower, compared to very hot environmental conditions in mid-summer, when generation times are generally shorter. The duration of the treatment windows should be adapted accordingly.
An example of insecticide treatment windows is provided in Figure 2. This example is based on different MoAs for an insect in an environment where the average temperature remains relatively constant for a period of five months, resulting in development times of 30 days per generation. Multiple applications of the same MoA can be done within a treatment window. When a treatment window is completed, a product with a different MoA should be selected for use in the following 30 days. If possible, a different MoA should even be applied in a third MoA treatment window. In the scenario described in Figure 2, five different MoAs are available (assuming all modes control the pest effectively) and several sprays of the same MoA may be possible within each spray window. It is essential that successive generations of the pest are not treated with compounds from the same MoA group (Figure 2). To account for this, and for overlapping generations of the pest within a cropping season, the same MoA cannot be repeated as a window treatment within a period of 60 days. Those individuals with genes for resistance to the insecticide applied in one window are unlikely to survive another MoA applied in the following window and can therefore not multiply to evolve into a resistant population.
Insecticide resistance is, however, managed best when an integrated pest management (IPM) strategy is followed. Depending on the scale of the farming system, pest species and the crop involved, an IPM strategy can include, amongst others, planting of pest-resistant cultivars, planting of trap crops, inundative releases of biological control agents, and the use of decision support systems such as action threshold levels to guide the use of pesticide. Most importantly, where insecticides are used, it should be done judiciously. This implies the following:
- Regular scouting to detect pests early.
- Applications should be need-based, and guided by action thresholds.
- Applications should be done at registered dosage rates.
- Application of insecticides should be done as window treatments.
- Insecticides with different MoAs should be rotated.
Resistance management of Bt crops
Resistance management of Bt crops is not based on rotation of MoA. Because the insecticidal toxin produced on Bt crop plants are produced in the plant from the seedling stage to crop maturity, it is not possible to rotate the MoA. IRM in Bt crops is based on the use of two components. Firstly, a high dose expression of the toxin inside the plant. This means that the dose of the toxin inside is sufficient to kill 99% of the target pest population. Secondly, refuges of non-Bt crop plants are planted where the target pest is not under selection pressure and where many pest individuals survive. Mating between the few survivors of the pest in the Bt crop area with the many survivors from the non-Bt refuge areas ensures that the proportion of the resistant individuals within a pest population remains low.
Poor performance of insecticides under field conditions
Poor efficacy of an insecticide at field level is sometimes incorrectly ascribed to pest resistance. There are many other factors that influence efficacy at field level.
Factors that contribute to increased risk of resistance evolution are the following:
- Common and indiscriminate use of insecticides due to overestimation of risk and a lack of action thresholds.
- Poor efficacy due to incorrect timing of insecticide applications.
- Applications to control the pest when the larvae are too large, leading to sub-lethal exposure.
- Low-volume applications.
Examples from South Africa
The issue of resistant pest populations is often raised by producers and the agro-chemical industry in South Africa. For example, the poor cutworm control in maize and Chilo partellus control in sorghum experienced recently was partly ascribed to resistance. There is, however, no evidence to support that these pests are resistant to insecticides. It is largely agreed that the increased pest status and poor control are due to changing farming practices. Since these pests occur in extensive farming systems, they are not routinely sprayed with pesticides. If control is applied, it is only done over
a short window, which reduces selection pressure for resistance evolution.
In intensive cropping systems where pesticides are routinely applied, for example on crops such as cabbage, tomato and potatoes, resistance may evolve more quickly. In such systems, pesticide resistance will in future escalate until all stakeholders evaluate their roles in resistance management and work together as a community to influence effective management. It is injudicious to refer to control failure as insecticide resistance without proper evaluation and scientific confirmation. It is therefore essential that training is provided to relevant stakeholders in the agrochemical industry to ensure that chemical control strategies are sound and that they do not promote resistance evolution. It is also of importance that cases of resistance evolution are recognised timely in order to apply mitigation measures such as insecticide mixtures, adaptations of the sequences of applications and rotations.
References
- https://irac-online.org/documents/irac-moa-group-30-irm-guidelines
- https://irac-online.org/documents/tutorial-on-moa-mechanisms/
- Panini, M, Manicardi, GC, Moores, GD & Mazzoni, E. 2016. An overview of the main pathways of metabolic resistance in insects. Invertebrate Survival Journal, 13:326 – 335.
- Van den Berg, J & Du Plessis, H. 2022. Chemical control and insecticide resistance in Spodoptera frugiperda (Lepidoptera: Noctuidae). Journal of Economic Entomology, 115:1761 – 1771.